High power lasers can be used to recreate conditions in the laboratory that can normally only be found off-planet, be it in the cores of Jovian planets, shocks of young supernova remnants, or in stellar interiors.
The Vulcan laser facility in particular is a versatile laboratory for performing WDM or lab-astro experiments. A range of different laser configurations, coupled to carefully designed targets and diagnostics, can be used to produce scaled-down versions of astrophysical objects and processes. The results of these experiments can then be compared to analytical and computational models to advance our understanding of the field.
Warm dense matter
Warm dense matter (WDM) is a state of matter that is typically characterised by densities from 0.1-10 times solid density and temperatures from 0.1 to 100 eV. Much of the physics in WDM depends on the ratio of coulomb energy to kinetic energy of the ion populations and is described as typically being strongly coupled with ECoulomb > EkT. In addition at high enough pressures (and lower temperatures) the plasma can become Fermi degenerate, creating large electron degeneracy pressures.
The equation of state (EOS) of a strongly coupled, degenerate plasma is not easy to formulate, and so experiments in the laboratory can provide crucial clues which will help us understand how the internal structure of such astrophysical objects are made up. By applying a pressure to a test material, how it reacts can be used to infer parts of the EOS. On a typical experiment this can be achieved by using one laser to launch a shock into a material to create WDM conditions, with a second probe laser used to generate an X-ray pulse that can diagnose changes in the structure of the material.
Astrophysical jets and Collisionless Shocks
By focusing powerful, pulsed lasers onto a solid target, huge temperatures and pressures can be produced in tiny, sub-mm volumes. The huge pressures in such a plasma lead to a rapid expansion of material or the creation of shockwaves in a very similar way to that which occurs in supernova remnants or jets produced by young stellar objects. Although an experiment in the laboratory occurs on much smaller spatial (1 mm vs 100s of light years) and temporal scales (1 ns vs 10,000s of years), a lot of the physics involved are scale invariant, permitting the reproduction of conditions that can be quantitatively tested rather than simply observed and computationally modelled.
The study of collisionless shocks, relevant to a range of astrophysical processes, has attracted a significant amount of attention in recent years. Due to the low density of astrophysical plasmas, the time between collisions is very large, hence they are considered collisionless, a condition that is hard to reproduce on earth, but achievable with high intensity laser-plasma experiments. Recent experiments at the CLF have studied conditions relevant to cosmic ray acceleration, or the origin of intergalactic magnetic fields.
Recent CLF Results
Experimental Observation of Thin-shell Instability in a Collisionless Plasma - H. Ahmed et al. Astro. J. Lett. 834 (2017)
The Vulcan laser was used to create a blast shell of collisionless plasma that could then be probed with an independent laser-accelerated proton beam. The proton radiography reveals the instability of the plasma shell, providing crucial experimental evidence for the nonlinear thin-shell instability (NTSI) process that could account for the density and flow patterns observed in Super Nova remnants.
Laser-plasma-based Space Radiation Reproduction in the Laboratory - B. Hidding et al.
Sci.Rep.
7 (2017)
Laser-accelerated radiation, produced on the Vulcan laser facility has been used to mimic space radiation in order to perform radiation hardness testing on electronic equipment. Unlike conventional accelerators, high power lasers can produce broadband radiation, very similar to that encountered in a space environment e.g. in the van-Allen belts. The work could pave the way for new testing procedures that could increase reliability of future space missions.
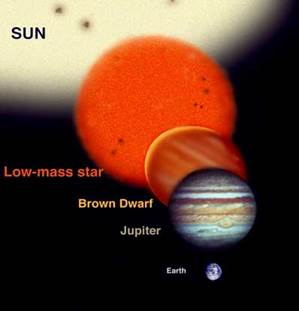 Credit: Gemini Observatory/Artwork by Jon Lomberg
| A team of researchers has used the Vulcan Petawatt laser to create unique conditions that are close to those at the centre of a brown dwarf. The work paves the way for better understanding of these hard to observe stellar objects. |
An experiment was conducted on the Vulcan laser to investigate turbulent amplification of magnetic fields, generated by colliding two jets of carbon plasma. The observation of strong non-linear growth of the magnetic fields provides important experimental evidence for the growth of galactic and intergalactic magnetic fields.
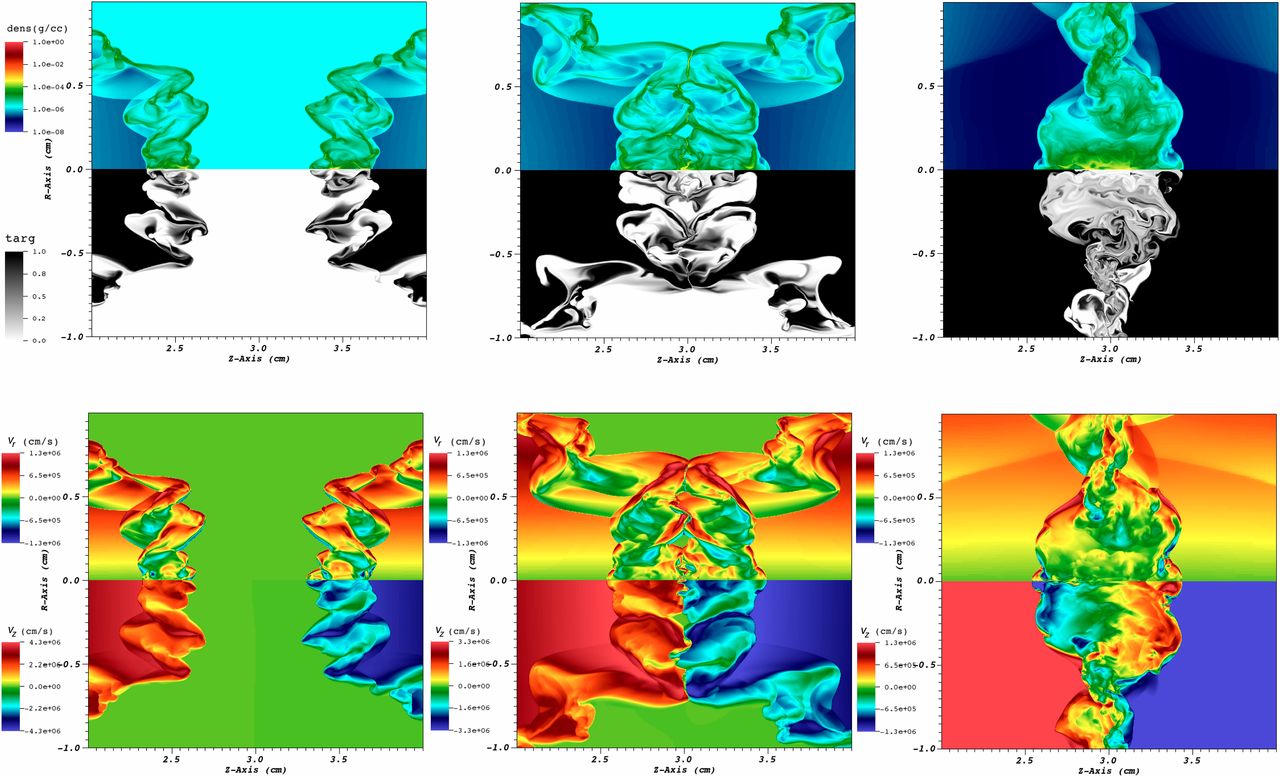
Credit: Meinecke et al. PNAS 112 2015
Terahertz Acoustics in Hot Dense Laser Plasmas (link opens in a new window)A Adak et al.
Phys. Rev. Lett.114 115001 (2015)
Turbulent amplification of magnetic fields in laboratory laser-produced shock waves (link opens in a new window)J Meinecke et al.
Nat. Phys.10 520-524 (2014)
Electron-Ion Equilibration in Ultrafast Heated Graphite (link opens in a new window)TG White et al.
Phys. Rev. Lett.112 145005 (2014)
X-ray scattering from warm dense iron (link opens in a new window)S White et al.
High Energy Density Physics 9 573-577 (2013)
Time-Resolved Characterization of the Formation of a Collisionless Shock (link opens in a new window)H Ahmed et al.
Phys. Rev. Lett. 110 205001 (2013)
Other resources