You get answers - and some really fast electrons; all of which has been chronicled in a recent paper, published in Physical Review Letters.
When completing their research, an international team of scientists from the Tata Institute of Fundamental Research (TIFR) in India, STFC's Central Laser Facility and Celia in France, had one question in mind; 'when fast electrons transit a solid, do they pass straight through the medium or do they stay within the material for a while instead?' Now, laser technology has provided answers.
By focusing a high power laser pulse on the surface of a glass target, the team were able to produce electrons that travelled at near-light speeds. Despite previous studies into hot-electron transport through solids, very little was previously known about the actual time that electrons spend inside a solid while dissipating their energy. Mainly, because understanding the transport of these electrons in the target involves a lot of complex physics. This research is therefore incredibly valuable for improving our understanding of the transport of energetic electrons through matter.
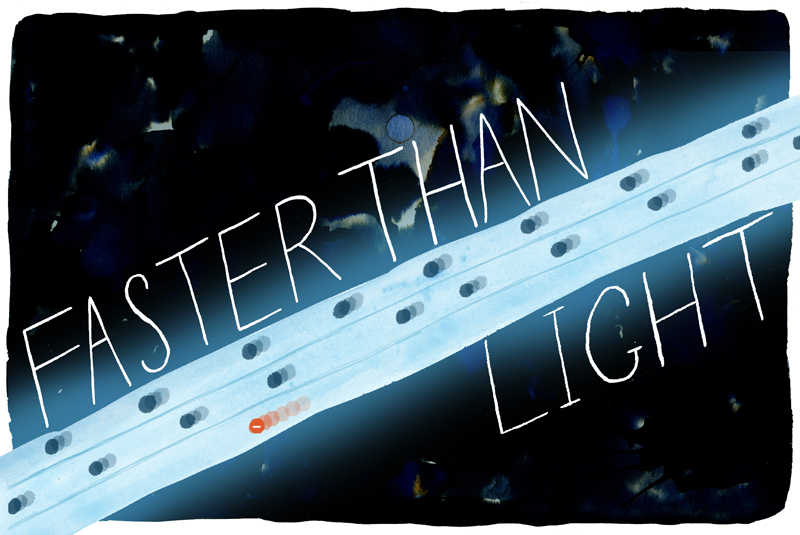
Figure 1: Ilustration by Helen Towrie, CLF
But why is this question so important? Well, things start to get really interesting when you consider that these electrons can drive giant current pulses (on the millions of ampere scale!) that are capable of generating some of the most exotic states of matter to ever be produced by man. We're talking mimicking conditions found in stars and the cores of planets, in a laboratory at RAL! Not only that but these incredible electrons are slap-bang in the centre of core technologies used to produce advanced X-ray, electron and ion sources for industrial and medical applications. So really handy then - but how do you generate these electron pulses in the first place?
The electron pulses in question were produced by a high power laser in TIFR (100 terawatt Titianium: Sapphire laser to be precise) irradiating a glass target which was hosted in a vacuum chamber on a table top (see figure 1 below). By focusing the laser beam to a micrometre spot, the team were able to create such a high intensity that electrons in the glass were instantly propelled from their positions and catapulted to speeds so fast that they approached the speed that light itself would travel in free space.
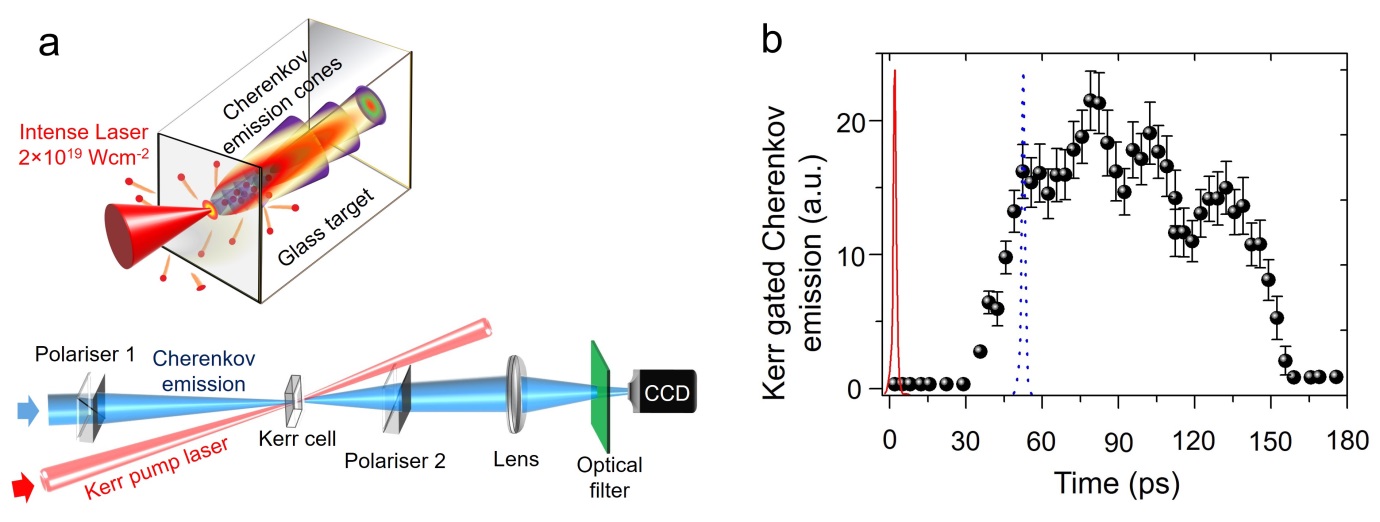
Figure 2: The table-top laser experiment at the Tata Institute of Fundamental Research, Mumbai.
(a) A high power laser pulse creates mega-ampere electron current pulses. Cherenkov emission in the form of cones is emitted by these electrons travelling faster than light in glass.
(b) the time trace for the Cherenkov emission measured in the experiment.
These electrons rush through the glass target behind. Inside glass, however, the light travels more slowly than in vacuum so some of these electrons are indeed faster-than-light. Scientists could then track these super-luminal electrons by measuring a special type of radiation - known as Cherenkov radiation - that is given off in this kind of process. The duration of the emission gives them an idea about the lifetime of these super-luminal electrons in the medium. Whilst normal electronic circuits can measure timescales as small as a billionth of a second, these measurements require much higher temporal accuracy. Therefore, the team used a part of the laser itself to generate an 'ultrafast shutter', lasting only two trillionths of a second, by driving a nonlinear medium. With this ultrafast shutter, the scientists were able to accurately measure the temporal evolution of the Cherenkov signal and thus the lifetime of these faster-than-light electrons with a thousand-times improved resolution. This conceded some fascinating results.
Moniruzzaman et al discovered that the electrons lasted much longer inside the solid than anticipated, had they been going straight through. Instead, the superluminal electrons stay around inside the solid for tens of picoseconds, continuously dissipating energy. That is over 1,000 times longer in duration than the electron pulses that originally entered the solid!
But how does this process work, you ask? The answer was discovered using some really nifty simulation technology, this time at CELIA (Centre Lasers Intenses et Applications) in France. Using this technology, the team discovered that multiple scattering events actually contributed to this "longer than expected stop-over". What this means is that if we can optimise the energy-exchange time of these fast electrons, scientists might be able to optimise laser-driven sources for an abundance of applications. Furthermore, if we can also get to grips with the lifetime of these fast electrons, we can unravel the mechanisms of their movement in solids, which is really key knowledge that can be applied to many areas of high-energy density science. This includes diverse fields such as laser-driven fusion and even the development of advanced radiation sources for industrial and medical applications. Very exciting indeed.
"This work was done through the Newton India collaboration that CLF has developed over the past few years", explains Rajeev Pattathil. "CLF is a major scientific contributor to this work as the idea of using this ultrafast gate (called Kerr-gate) to measure the emission was jointly developed by myself and TIFR scientists. In fact, the very first idea of using a Kerr-gate for measuring plasma emissions was proposed in a paper published a few years ago by CLF scientists."
The research was supported by the Department of Science and Technology, Ministry of Science and Technology, Government of India; and the Newton-Bhabha UK-India programme. The full publication is available to view in Physical Review Letters.
Life in the fast lane is really starting to pick up a lot more pace - fasten your seatbelts!